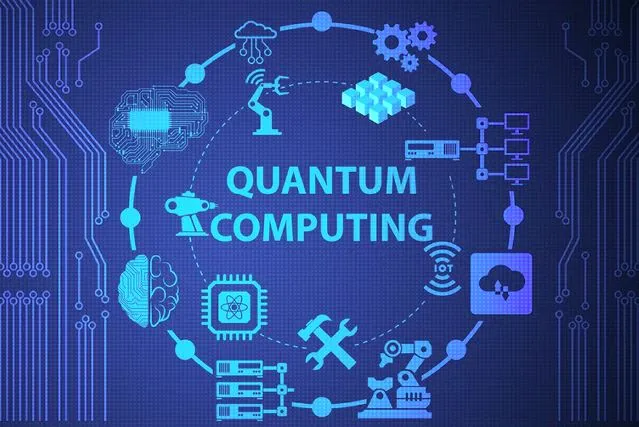
What is Quantum Computing with Example and Implications
In the realm of modern technology, quantum computing stands as a beacon of potential, promising to revolutionize computation as we know it. Unlike classical computers, which use bits as the fundamental unit of information represented as either 0 or 1, quantum computers utilize quantum bits, or qubits. These qubits can exist in states of 0, 1, or any superposition of these states, exponentially expanding the computational power and allowing for new approaches to problem-solving that were previously inconceivable.
What is Quantum Computing?
These unique properties enable quantum computers to tackle problems that are computationally intensive or even intractable for classical computers. For instance, optimization tasks, like route planning or financial portfolio management, benefit from quantum computing’s ability to explore vast numbers of possibilities simultaneously. Moreover, quantum cryptography promises unbreakable encryption methods through the use of quantum key distribution protocols, leveraging the principles of quantum entanglement to ensure secure communication.
The development of practical quantum computers faces hurdles like maintaining qubit coherence and minimizing errors inherent in quantum systems. Progress in overcoming these challenges is crucial for realizing the full potential of quantum computing across various fields. As researchers worldwide strive to harness quantum mechanics for computational purposes, the landscape of computing is poised for a transformative shift, where quantum algorithms and applications may redefine what is achievable in science and technology. Quantum computing stands not only as a promising technological frontier but also as a testament to humanity’s ongoing quest for deeper understanding and mastery of the natural world at its most fundamental levels.
Principles of Quantum Mechanics in Computing
Superposition: Unlike classical bits that are definite (either 0 or 1), qubits can exist in a superposition of states. This means a qubit can represent both 0 and 1 simultaneously until measured. This property allows quantum computers to explore multiple potential solutions to a problem simultaneously, significantly speeding up certain types of calculations.
Entanglement: Qubits can be entangled, meaning the state of one qubit instantaneously influences the state of another, regardless of the distance between them. This phenomenon enables quantum computers to perform parallel computations in an unprecedented manner. By leveraging entanglement, quantum algorithms can solve complex problems more efficiently than classical algorithms, especially those involving vast amounts of data or interconnected variables.
Quantum Interference: Qubits can interfere with each other, allowing quantum computers to process information in ways that exploit interference patterns. This capability leads to faster and more efficient computations for specific tasks such as optimization and simulation. Quantum interference enables quantum algorithms to amplify correct answers and suppress incorrect ones, enhancing the overall computational efficiency and accuracy.
Quantum Computing Example: Quantum Supremacy
Quantum supremacy marks a pivotal achievement where a quantum computer surpasses the computational capabilities of even the most powerful classical supercomputers for a specific task. Google’s 2019 experiment, using a quantum processor named Sycamore, demonstrated quantum supremacy by solving a problem in just 200 seconds that would take classical supercomputers thousands of years. This milestone showcases the potential of quantum computing to tackle complex problems exponentially faster than classical methods.
Quantum Computing Illustrative Example
Consider a complex optimization problem, such as finding the lowest energy state of a molecule—a task critical in drug discovery and material science. Classical computers struggle with the exponentially growing computational demands of such problems as the size of the molecule increases. Quantum computers, on the other hand, can model quantum systems directly, leveraging quantum mechanics to explore multiple potential solutions simultaneously through superposition and entanglement. This capability could revolutionize fields like pharmaceuticals by accelerating the discovery of new drugs or materials with specific properties.
Quantum Computing Challenges and Future Outlook
While quantum computing holds immense promise, significant challenges persist. Maintaining qubit stability and developing error correction mechanisms are critical for scaling quantum systems to handle larger, more complex computations reliably. Moreover, integrating quantum algorithms into practical applications requires overcoming technical hurdles and ensuring compatibility with existing infrastructure.
Looking forward, continued research and collaboration across academia, industry, and government sectors are crucial for advancing quantum computing. As advancements in hardware, algorithms, and applications accelerate, quantum computers may soon unlock solutions to problems that are currently intractable for classical computers. The future of quantum computing promises not only faster computations but also transformative impacts across scientific research, cryptography, artificial intelligence, and beyond.
Quantum Computing Future Applications
As quantum computing continues to advance, its potential applications span across diverse industries and scientific disciplines, promising to revolutionize various fields:
Cryptography and Cybersecurity
Quantum computing poses both a threat and an opportunity for cybersecurity. On one hand, quantum computers have the potential to break widely used cryptographic algorithms, such as RSA and ECC, which currently secure sensitive data and communications. This capability could render current encryption methods obsolete, necessitating the development of quantum-resistant encryption techniques. On the other hand, quantum cryptography offers secure communication channels based on the principles of quantum mechanics, leveraging quantum key distribution (QKD) to provide theoretically unhackable encryption.
Drug Discovery and Materials Science
Quantum computers excel in simulating molecular interactions and properties, offering a quantum leap in computational chemistry. Traditional methods for drug discovery and materials science involve complex simulations that require enormous computational resources. Quantum computers can accurately model quantum systems, allowing researchers to explore new drug candidates, understand molecular behavior with unprecedented detail, and design advanced materials with tailored properties. This capability has the potential to accelerate the discovery of new drugs, optimize chemical processes, and revolutionize material design.
Quantum Computing Optimization Problems
Quantum algorithms are particularly adept at solving optimization problems that are challenging for classical computers. These include tasks such as route optimization for logistics, scheduling, financial portfolio optimization, and resource allocation. Quantum computers leverage quantum parallelism and interference to explore multiple solutions simultaneously, significantly speeding up the process of finding optimal solutions to complex problems. This capability can lead to more efficient transportation networks, better financial strategies, and optimized resource utilization across various industries.
Quantum Computing Artificial Intelligence (AI)
Quantum computing holds promise for enhancing AI capabilities by accelerating complex calculations involved in machine learning algorithms. Tasks such as training deep neural networks, processing large datasets, and conducting sophisticated pattern recognition can benefit from the quantum speedup. Quantum machine learning algorithms may enable AI systems to achieve greater accuracy and efficiency, unlocking new applications in areas such as image and speech recognition, natural language processing, and autonomous systems.
Climate Modeling and Energy Optimization
Quantum computers have the potential to revolutionize climate modeling and energy optimization by enabling more accurate simulations and predictions. Climate models require vast computational resources to simulate complex interactions between atmosphere, oceans, land, and ice. Quantum computers can enhance the accuracy and resolution of these models, providing insights into climate change dynamics, extreme weather events, and environmental impacts. Additionally, quantum algorithms can optimize energy production and distribution systems, leading to more efficient renewable energy technologies, smarter grid management, and sustainable resource management strategies.
Quantum Computing Socio-Economic Implications
The advent of quantum computing is poised to bring about profound socio-economic implications:
Job Creation and Industry Transformation: The development and commercialization of quantum technologies will create new job opportunities in research, engineering, software development, and specialized industries. Existing sectors may undergo transformation as quantum computing applications become integrated into their operations.
Global Competitiveness: Countries and companies investing in quantum computing research and development stand to enhance their technological capabilities and global competitiveness. The race for quantum supremacy and leadership in quantum technology could shape geopolitical dynamics and economic landscapes.
Ethical and Regulatory Challenges: Quantum computing raises ethical considerations related to data privacy, cybersecurity, and the responsible use of powerful computational capabilities. Regulatory frameworks will need to evolve to address these challenges and ensure the safe and ethical deployment of quantum technologies.
Conclusion
In conclusion, quantum computing represents a paradigm shift in computation, leveraging the peculiarities of quantum mechanics to solve problems that are currently intractable for classical computers. As research and development in quantum computing advance, we can expect transformative changes across various industries, paving the way for a future where quantum computers play a central role in tackling some of humanity’s most challenging problems.
The journey towards practical quantum computing is ongoing, with numerous exciting discoveries and advancements on the horizon. As we unravel the mysteries of quantum mechanics, the potential for quantum computing to reshape our world becomes increasingly clear, promising a future where the limits of computation are defined not by the capabilities of classical machines, but by the quantum possibilities that lie ahead.